Self Assembly in Microemulsions and Aqueous Electrolytes
Self-assembly is a general phenomenon where components spontaneously organize into ordered structures, and this is particularly significant in multicomponent solutions. In systems like oil and water microemulsions, surfactants act to stabilize immiscible phases, leading to the formation of organized structures such as reverse micelles. These reverse micelles, with a water core surrounded by surfactants in an oil phase, are key in various industries, especially in liquid-liquid extraction (LLE), where they facilitate the selective solubilization and transport of analytes like metal ions. This behavior is further controlled using phase modifiers, which help prevent phase separation and optimize extraction processes in industrial applications.
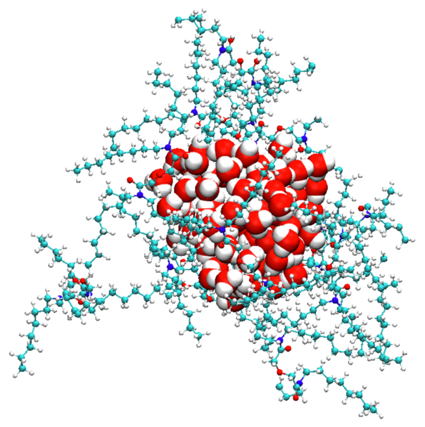
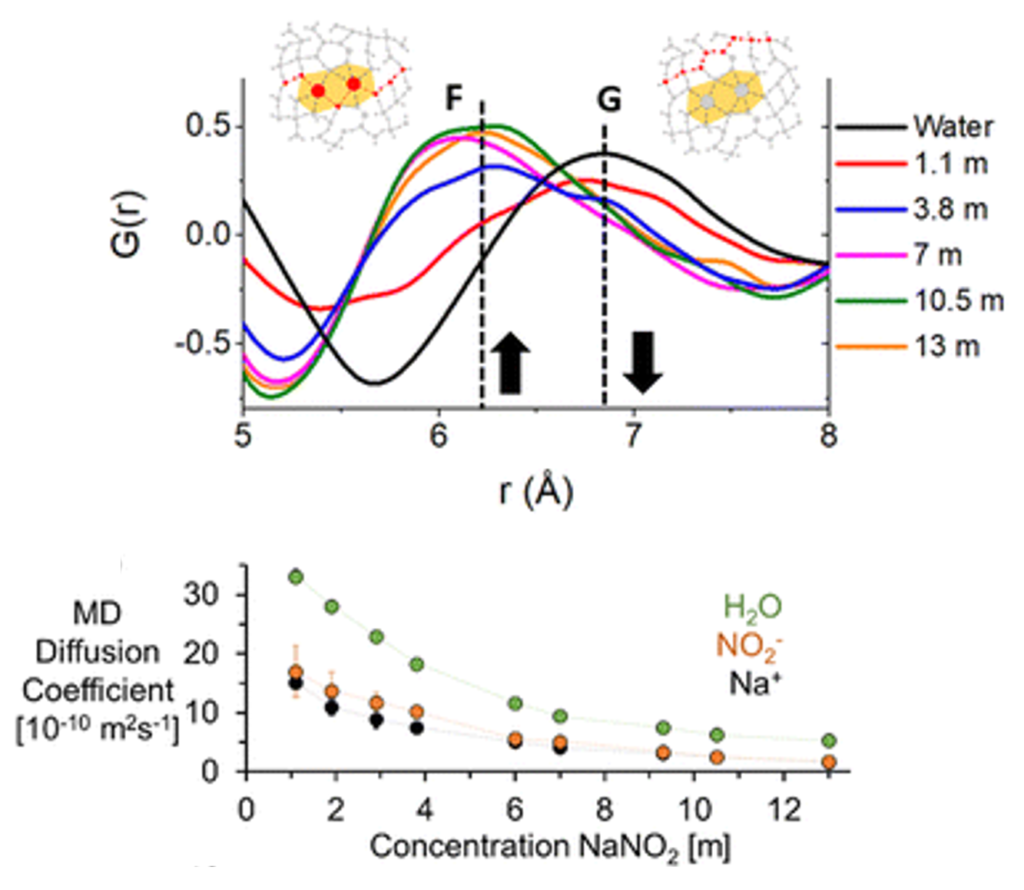
Concentrated Aqueous Electrolytes
In extremely concentrated aqueous electrolytes, the so-called “water-in-salt” solution, ions may aggregate into clusters or networks. Understanding the composition of ion clusters, the linking types of building blocks, and the interconversion dynamics between cluster macrostates is essential for probing into the solution self-assembly mechanism, such as the stability of ion clusters and nucleation theory.
The Speciation-Transport Paradigm
Untackling the relationship between ion speciation and solution transportational behaviors, such as Fick’s law of diffusion and Newton’s law of viscosity, has been investigated for many decades, from either empirical or ab initio approaches. In our group, we would like to create a modified quantitative theory to correlate the dynamics of local and extended ion-water networks with macroscopic transportational collective variables, which exhibits ion-specific concentration-dependent trend.
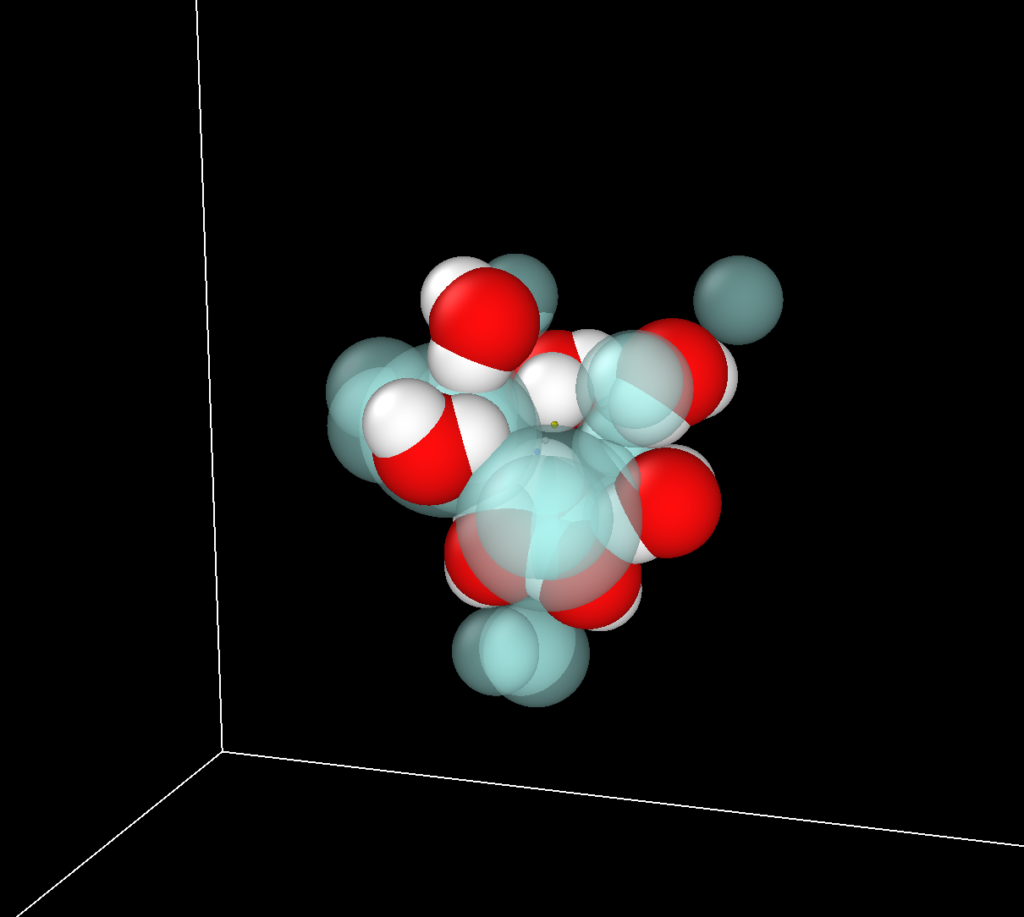
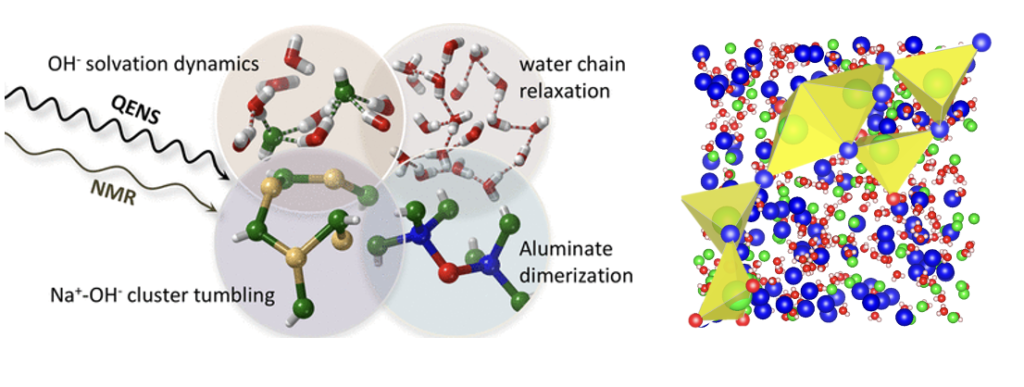
Multiscale Motion
Clustering/aggregation or ion-network formation are well established in concentrated aqueous electrolytes. Rotational timescale of water is altered when ions aggregate. Studies in sodium aluminate solutions using QENS and 1H NMR experiments have shown that water and ion clusters exhibit localized motions on timescales from 40-750 ps, with slower, collective dynamics emerging as concentration increases. We use Molecular dynamics simulations to complement experimental findings, to look into ion networks and clustering that couple the motion of water to large ion clusters.