Motivation for Separations
Separation science is critical for a wide array of technologies and industries, and it is essential to meeting societal needs. Chemical separation processes are used to divide chemical mixtures into their constituent elements, usually to enrich a product stream or to obtain pure components. Separations are needed to extract natural resources for energy storage and use. Furthermore, optimizing separations allows for the recovery of valuable materials and minimizes waste. Our group emphasizes studying liquid-liquid extraction through the use of tools such as molecular simulations and data science techniques. In particular, we are interested in how the dynamics and structure of liquid-liquid interfaces affect mass transfer.
Pictured here is an electron-density profile (red line) obtained by x-ray reflectivity data and a molecular dynamics simulation to construct a model of the ordered arrangement of amphiphilic DHDP molecules. (Source: NASEM Separation Report)
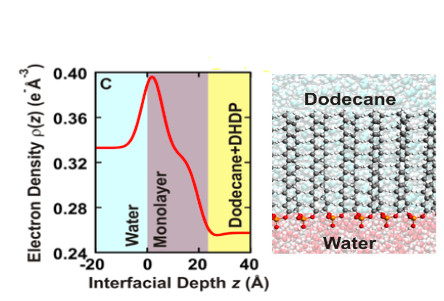
Microsolvation and Interfacial Properties
Interfacial properties at the liquid interface are an area of interest for biological, chemical, and environmental implications. These properties range from the dynamics of solvent molecules at the interface to the hydrogen bonding networks of interfacial water molecules (using our in-house chem-networks code).
In addition, our group has studied the interfacial properties, such as surface tension, and the trend with the distribution and persistence of microsolvated species at the interface. Here, we showed that while surface tension is sensitive to the specific alkane isomers present, the microsolvation properties are not. This has important implications for the underlying organization and mechanisms that lead to the formation of the interface.
Here is an example of two possible types of adducts that can form at the liquid-liquid interface between water and a common surfactant, Tributyl phosphate (TBP). On the left, two TBP form a water bridge dimer. On the right, an adsorbed TBP binds to two interfacial water molecules. These conformations lead to different interfacial structures.
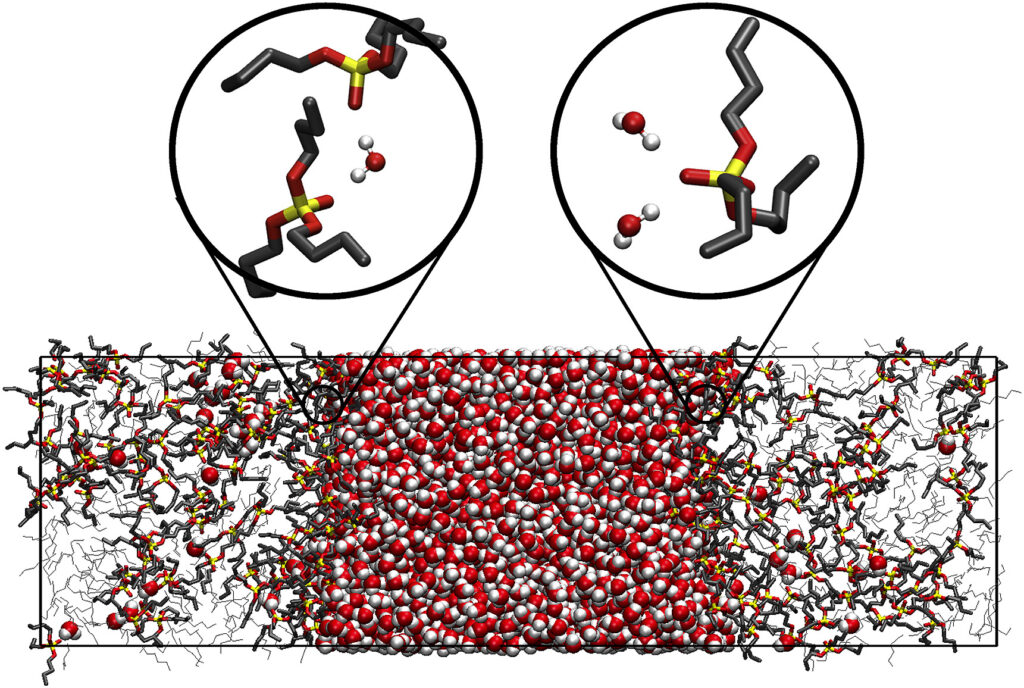
Surfactant Structure at the Liquid-Liquid Interface
The self-organization of surfactants at the liquid-liquid interface can be affected directly by interfacial roughness, large fluctuations, defects at the interface, and adsorbate-adsorbate interactions. As a result, understanding the direct impact of these effects on self-assembly is crucial to understanding interfacial properties and mass transfer.
Our group has offered a unique perspective by using sublevel-set persistent homology to characterize the surface active amphiphile self-assembly under different conditions. Our method provides a generalized approach to studying these effects, allowing it to be applied to the most extreme interface cases. In addition, our methods can be applied to computer simulation and experimental images.
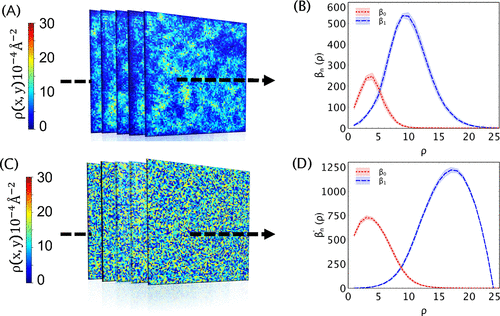
Mass Transport across the Liquid-Liquid Interface
Mass transport across the liquid-liquid interface (LLI) is essential for a range of applications, from the transport of drug molecules across biological interfaces to the reprocessing and extraction of metals from solutions. We aim to study this process to understand the transport mechanism and the associated rates.
Our group has identified two transport mechanisms through MD simulations. The first is called the primary transport mechanism, in which the presence of surfactant molecules leads to adducts forming with interfacial molecules, decreasing other intermolecular forces at the LLI. This leads to the formation of a protruding structure, which can detach from the interface, resulting in mass transfer and the formation of a reverse micelle.
In addition, we also have identified the secondary transport mechanism, also called the “door hinge mechanism”. Here, organic surface-active molecules such as octanol form large organized bi-layers. Molecules on the sides of the bi-layers will form an adduct with interfacial aqueous molecules or ions. The adduct then swaps its orientation to the other side of the bilayer, leading to a reversible mass transfer process.
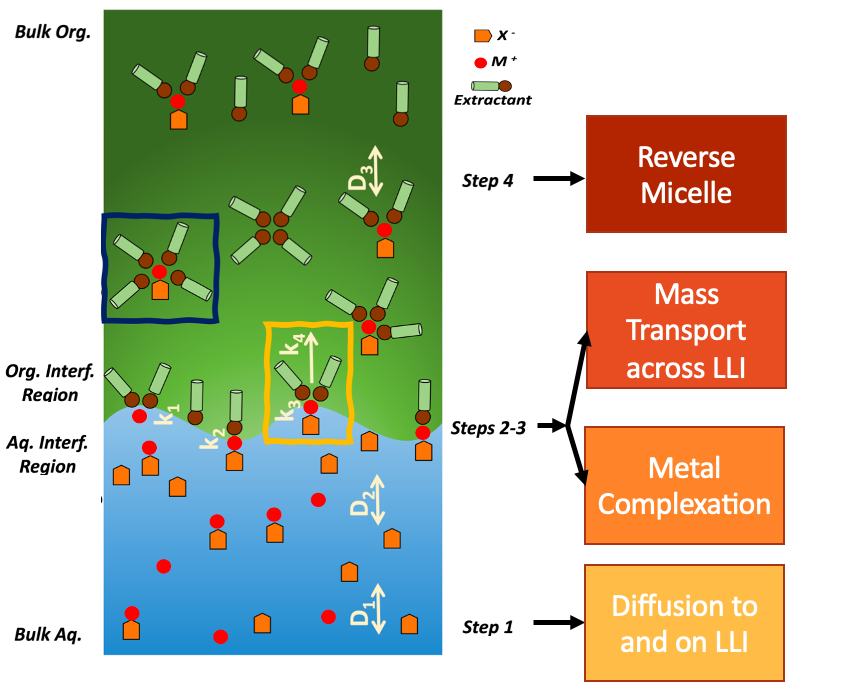